Remembering the Harmful Effects of Ionizing Radiation
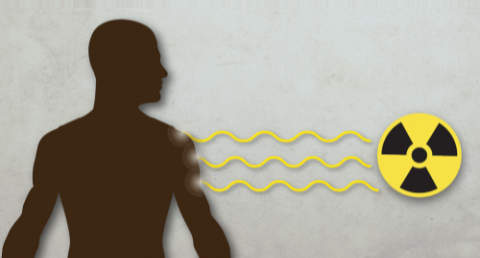
Remembering the Harmful Effects of Ionizing Radiation Introduction Scientists have known for a very long time now that exposure to ionizing radiation can have harmful effects in humans. Awareness first manifested itself by radiation burns on the early experimenters who paid dearly for their work. Fortunately, modern radioactive devices employ many well engineered safety measures […]
A Watered-Down Perspective to Radiation Measurement Units
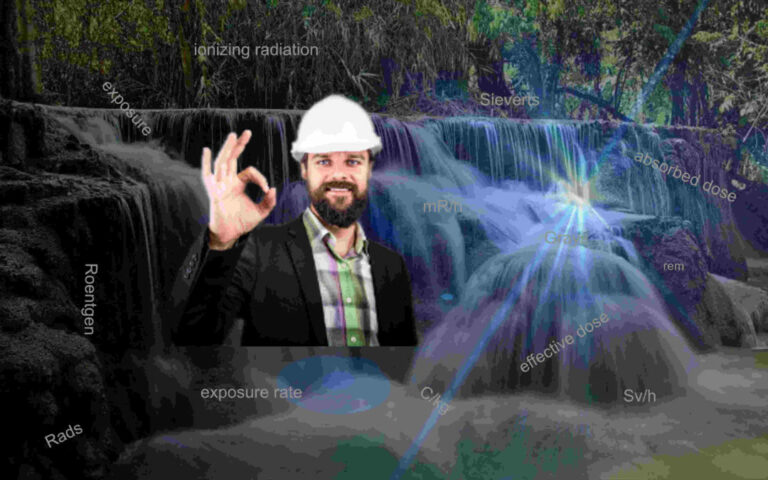
Relating Radiation Measurement Units to Water If I were attempting to explain radiation measurement units to something even a child could understand, I would liken it to water which I feel really works well as a metaphor. In many ways one can draw simple analogies using water to relate it to radiation. After completing my […]